A suggestion to perform ‘First Fire’ at La Porte, TX based on an analysis of Recuperator of ALLAM Cycle Mohideen Ibramsha 1968 Alumni of Thiagarajar College of Engineering, Madurai, TN, India 1974 intellectual son of PhD guide Prof. V.Rajaraman & Mrs. Dharma Rajaramn, CS, EE, IIT, Kanpur, UP, India 1991 First HOD of CSE, CEC [now BSAU] Chennai, TN, India Associate Professor (Retd), Computer Science, Framingham, MA, USA Consultant R&D, M A M College of Engineering, Trichy, TN, India Advisor, HyDIGIT Pte Ltd, Singapore Email: ibramsha7@yahoo.com
This article was posted elsewhere on Monday, April 16, 2018 - 01:02 pm: It is re-posted here without altering the contents. Introduction: The ALLAM Cycle is a game changer. The implementation details of the power plant are detailed by the inventor of ALLAM Cycle, Rodney Allam and his co-authors found at https://www.sciencedirect.com/science/article/pii/S187661021731932X We use the data given in the PDF for our analysis of the 300 MWe ALLAM power plant. Heating flows to 717 C at 310 bar is beyond the PCHE For ALLAM Cycle Heatric has delivered the heat exchanger. The heat exchanger characteristics are described in the following Youtube video. https://www.youtube.com/watch?v=B92enSIvVE8
===
At 1:14 of 4:04 video
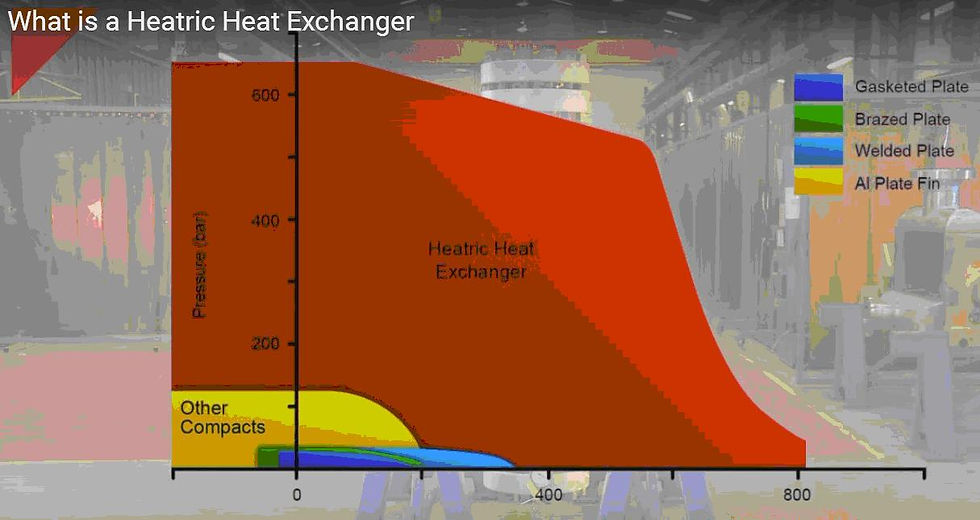
=== The above Youtube video was uploaded on December 12, 2013. The following URL https://www.heatric.com/index.html was accessed on March 17, 2018. The video to introduce Heatric happens to be the same video as the Youtube video above. Using the pencil function in the ‘Paint’ program, we find the following pixel coordinates.
Origin X 367; Y 581
400 bar pressure X 367; Y 271
800 C temperature X 989; Y 581
Highest temperature and maximum pressure at that temperature X 998; Y 546 The highest temperature has X pixel value of 998. The temperature is (998 – 367)/(989 – 367) x 800 = (631/622) x 800 = 811.58 C. The corresponding pressure is (581 – 546)/(581 – 271) x 400 = (35/310) x 400 = 45.16 bar.
Flow 13 in the description leaving the Recuperator has a temperature of 717 C at a pressure of 310 bar. The pixel for 310 bar pressure is 271 + (581 – 271)/400 x (400 – 310) = 271 + 310/400 x 90 = 271 + 69.75 = 340.75 approximated to 341. On the curve, we get X – 857 corresponding to Y = 341. The maximum temperature for 310 bar pressure is (857 – 367) / (989 – 367) x 800 = (490/622) x 800 = 630.23 C. Thus given the performance as per the curve, for a pressure of 310 bar, the flow would have a maximum temperature of 630 C and not 717 C. The diagram has a straight line also. On the straight line we get X = 678 corresponding to a temperature of (678 – 367)/(989 – 367) x 800 = (311/622) x 800 = 400 C. Being conservative, we could take a temperature of 400 C at 310 bar pressure. Likewise for flow 9 also we take the temperature to be 400 C. As per the performance of the Heatric PCHE given by Heatric in their web site, we could not use the Heatric heat exchangers to increase the temperature of the flows to 717 C once the pressure is at 310 bar. If the pumps that increase the pressure of flows 8 and 12 are instead used to increase the pressure of the flows after the heat exchanger, the Heatric heat exchanger could heat the flows to 717 C. Flow 12 also is compressed to 310 bar before entering the Recuperator. We find that flow 12 before getting compressed to 310 bar has a pressure of 99 bar. The pixel value for 99 bar is 581 – (581 – 271)/400 x 99 = 581 - (310/400) x 99 = 581 – 76.725 = 504.275. On the curve we get a temperature corresponding to pixel value 935. The corresponding temperature is (935 – 367)/(989 – 367) x 800 = (568/622) x 800 = 730.55 C. On the straight line, for 504 we get temperature for pixel value 839, which is (839 – 367)/(989 – 367) x 800 = (472/622) x 800 = 607.07 C. We need not be conservative as discussed above in view of Figure 12 in https://inldigitallibrary.inl.gov/sites/sti/sti/3901047.pdf which shows that as of 2003 itself PCHE was used as ‘feed/ effluent exchanger for mini refinery.’ Hence we conclude that Heatric heat exchanger used in the La Porte plant delivers flows 9 and 13 at 717 C but at 100 bar instead of at 310 bar. We need to pump these flows to higher pressures. Increase pressure of flows after PCHE with reciprocating pump A review of compressors at http://www.purchasing.com/construction-equipment/air-compressors/air-compressor- comparison/ Shows that reciprocating compressors alone offer pressures of 300 bar. The article “A review of Reciprocating Compressor Crosshead Pin Reversals” by Mr. Dwayne A Hickman is published in COMPRESSORtech in pages 32 to 38 of the April 2013 issue. In an advertisement that appears in page 33 of that issue, we find the following URL accessed on March 19, 2018.. https://www.burckhardtcompression.com/solution/compressor-technologies/process-g as-compressor-api-618/ === Burckhardt Compression provides a robust and reliable Process Gas Compressor of Swiss quality designed for lowest life cycle costs. The design is according to individual application requirements, customer specifications and complies with the API 618 5th edition guidelines. It is available oil-free or lubricated, horizontal or vertical. It handles easily hydrogen, hydrocarbons and corrosive gases at high pressure. Burckhardt Compression has over 160 years of experience in manufacturing reciprocating compressors and offers a worldwide one-stop service. … Discharge pressure [bara] / [psia] up to 1000 / 1500Oil-free up to 300 bara or lubricated up to 1’000 bara === The cut out of the compressor shown has two pistons, one in compression and the other in suction, and thus it is possible that the problem of ‘non-reversal of piston’ discussed in the 2013 article does not occur with this compressor. As the compressor is oil free up to 300 bar, we suggest that the ALLAM Cycle combustor and turbine be operated at 290 bar or less pressure to use the available reciprocating compressors in the market. It is expected that reducing the pressure from 300 bar to 290 bar would not affect the operation of the turbine, leading to just a marginal reduction in electricity generation. As of now, as a first step, we pass the oxidant [ flow 12 ] without compression through the Heatric heat exchanger getting flow 12A at 99 bar with a temperature of 717 C. We compress this flow adiabatically using an appropriately designed reciprocating compressor to flow 13 with a pressure of 300 bar for the ALLAM Cycle. We analyzed the ALLAN Cycle flows for consistency and changed some flows. The consistent flows are given in https://mohideenibramsha7.wixsite.com/website/single-post/2018/08/02/Hydrogen-Economy-2 For convenience their description is given below.
1 Turbine Exhaust is 923 Kg/s at a temperature of 727 C and a pressure of 30 bar.
2. At a temperature of 43 C, the ‘Cooled Turbine Exhaust’ is 564 Kg/s with a pressure of 29 bar. The ‘2A Ancillary Bypass Flow ‘ is 337.5 Kg/s with a pressure of 29 bar and a temperature of 32.8 C. [The balancing equation is 923 = 564 + 337.5 + 21.5 of H2O removed from the Recuperator and discarded.]
3. Dry CO2 is at a pressure of 29 bar, a temperature of 17 C and is 563 Kg/s.
4. Compressed CO2 is 900.5 Kg/s after subtracting the 22.5 H2O removed at the Recuperator from the 923 Kg/s of the Turbine Exhaust which is CO2 plus steam.
5. Circulating CO2 is 873 Kg/s at 100 bar and 23 C.
6. CO2 from burnt fuel is 27.5 Kg/s at 100 bar and 23 C. The sum of ‘Circulating CO2’ and ‘CO2 from burnt fuel’ equals 900.5 Kg/s corresponding to the CO2 in the Turbine Exhaust.
7. Cold Circulating CO2 is 873 Kg/s at a temperature of 16 C and 100 bar pressure.
8. The ‘Cold Circulating CO2’ is split into ‘Cold Circulating CO2 part’ of 683.5 Kg/s and ‘CO2 for adding Oxygen’ of 189.5 Kg/s. The ‘Cold Circulating CO2 part’ enters the Recuperator and is withdrawn in two flows of ‘Hot Circulating CO2’ after four stages of the Recuperator and as ‘Turbine Cooling Flow’ after three stages of the Recuperator. The amount of ‘Turbine Cooling Flow’ is dependent on the load on the turbine and is denoted as X Kg/s. The sum of the ‘Hot Circulating CO2 part’ and the ‘Turbine Cooling Flow’ must equal 683.5 Kg/s. Thus the 8A – Hot Circulating CO2 is (683.5 – X) Kg/s at 100 bar and 717 C.
9. ‘Hot Circulating CO2’ is at a temperature of 717 C at a pressure of 300 bar. It is (683.5 – X) Kg/s where X Kg/s is the amount of ‘Turbine Cooling Flow.’ Flow 8A leaves the Recuperator at a temperature of 717 C at a pressure of 100 bar. This low pressure flow is compressed by a specially designed reciprocating pump to a pressure of 300 bar. The quantity is decreased as the ‘Turbine Cooling Flow’ leaves the entered Flow 8 after 3 stages of the heat exchanger. Flow 8A becomes flow 9 after the compressor. The flow 9A, Turbine Cooling Flow is X Kg/s at 100 bar and 548.5 C. With Flow 9 at 586 Kg/s the ‘9A Turbine Cooling Flow’ becomes 683.5 – 586 = 97.5 Kg/s. The ‘9A Turbine Cooling Flow’ varies with load. When the ‘9A Turbine Cooling Flow’ changes, Flow 9 also changes. To clarify this inter dependence between flow 9 and ‘9A Turbine Cooling Flow’ we have used the variable X. The ‘9A Turbine Cooling Flow’ is compressed after it leaves the Recuperator to 300 bar and becomes ‘9B Turbine Cooling Flow’
10. ‘CO2 for adding Oxygen’ is 189.5 Kg/s at 199 bar pressure and 16 C temperature.
11. ‘Oxygen’ is 40 Kg/s at 16 C temperature and 100 bar pressure.
12. ‘Oxygen added CO2’ has a pressure of 99 bar and 228.5 Kg/s. The temperature is given as 2 C which is possibly a typo. We ignore this temperature. After passing through the Recuperator, ‘12A Hot Oxygen added CO2’ has a flow of 229.5 Kg/s, a pressure of 99 bar and a temperature of 717 C.
13. ‘Compressed Oxygen added CO2’ called ‘Supplemental Oxygen’ is 229.5 Kg/s with a temperature of 717 C at a pressure of 300 bar.
14. The ‘Gaseous Fuel CH4’ is 10 Kg/s at a pressure of 330 bar and a temperature of 266 C.
The above changes could be incorporated in the design of the 300 MWe plant.
Two PCHEs in alternating mode We investigate the possibility of replacing the first stage of the Recuperator that receives the Turbine Exhaust by two Heatric PCHEs receiving the Turbine Exhaust during alternating periods. In one period the PCHE A receives the Turbine Exhaust and Flow 9, while PCHE B has Turbine Cooling Flow alone passing through it cooling PCHE B. PCHE A heats up the Flow 9 to 717 C at 310 bar such that no deformation occurs in PCHE A. The time required to diffusion bonding of two plates under pressure is given at https://en.wikipedia.org/wiki/Diffusion_bonding This time is dependent on M, A, D, and dC/dx, where M is mass, A is area, D is diffusion coefficient, and dC/dx is the concentration gradient between the two surfaces to be bonded. The bonding time is inversely proportional to D and dC/dx. The diffusion bonding is carried out between 50% - 70% of the absolute melting temperature of the materials. For the steels used in PCHE, 316 and 316L the absolute melting temperature is given as 1375 – 1400 C in https://www.bssa.org.uk/topics.php?article=103 Thus a temperature of 717 C is very close to the 50% of the absolute melting temperature of the PCHE material. The pressure applied for bonding is identified now. From slide 8 of 46 of https://www.slideshare.net/Arunnegemiya/diffusion-bonding-71482843 we find that for diffusion bonding of steel, the temperature and pressure required are about 1150 C and 700 Kg/cm2. As 1 Kg/cm2 is equal to 98.0665 kilo Pascal, the pressure is 700 x 98.0665 kilo Pascal. One kilo Pascal is 0.00986923 bar. Thus the pressure for diffusion is 700 x 98.0665 x 0.00986923 bar = 700 x 0.96784084 bar = 677.49 bar. The concentration gradient is useful when considering the diffusion bonding of the Heatric PCHE plates. Our concern is to avoid deformation of the flow pipes inside the Heatric PCHE when the flows are 30 bar and 717 C from the Turbine Exhaust and 310 bar and 717 C for flow 9. The pressure of flow 9 is less than 45% of the pressure required for bonding and thus there should be no deformation in the PCHE. What should be dC/dx to avoid deformation of the flow pipes at 717 C and a pressure of 310 bar? Under deformation, the high pressure flow would push the low pressure flow pipe. Since the temperature is substantially less than the absolute melting temperature, we do not expect any deformation to occur. We would have no concern if the temperature was 600 C or less in view of the following. https://www.heatric.com/hres/2009_may%20dave%20southall.pdf === Heatric carried out the following trials under this test programme: 1. Room temperature tensile testing. 2. 100 hour creep and stress rupture testing, at a temperature of 600 C. 3. 1000 hour creep and stress rupture testing, at a temperature of 600 C. … TABLE V Test Duration and % TPS results for 1000 hour creep tests, at a temperature of 600 C and an applied stress of 185.0 N/mm², for welded SA240 (stock codes W1, W2, W3), diffusion-bonded SA240 (stock codes B1, B2, B3), and as received SA240 (stock coded A1, A2, A3). … Again, for each stock code that was creep tested, a further four samples from the same stock code were stress rupture tested. All of these tests were discontinued after 110% of the required test time of 1000 hours, without any failures. === The pressure applied is 185.0 N/mm2, which is 185.0 x 10 bar. As the pressure applied was 1,850 bar about 6 times the 310 bar for the flow 9, the pressure is within range. However, the temperature of flow 9 is 717 C is more than the 600 C tested causing concern. Alloy 617 is approved for use up to 982 C as reported in the article below. https://www.heatric.com/hres/alloy%20617%20for%20the%20high%20temperature%20diff usion_bonded%20compact%20heat%20exchangers.pdf === The design of high temperature heat exchangers will be influenced by the alloy’s physical properties especially thermal conductivity and thermal expansion. Temperature changes within heat exchangers under transient conditions such as start-up and shut-down can generate thermal stresses which may eventually initiate and propagate fatigue cracks. These thermal stresses are generally proportional to the alloy’s thermal expansion coefficients. A suitable heat exchanger material should have a high thermal conductivity and low rate of thermal expansion. From Table 1, we find that alloy 617 has thermal conductivity of 28.7 W/mK, and coefficient of expansion of 16.3 μm/m C. From Figure 1, we find that the maximum allowable stress decreases from about 145 MPa at about 625 C to about 5 MPa at about 980 C ===
How does stainless steel compare with alloy 617? From http://www.mace.manchester.ac.uk/project/research/structures/strucfire/materialI nFire/Steel/StainlessSteel/thermalProperties.htm we find that stainless steel has about 23 W/mK at 700 C and thermal elongation of about 12.5 x 10-3 at 700 C, giving a coefficient of expansion of 17.86 μm/m C. We conclude that stainless steel is comparable to alloy 617. We cannot use a PCHE made of alloy 617 in ALLAM Cycle, as well as in CAM ALLAM Cycle, because of the following reasons. https://www.sciencedirect.com/science/article/pii/0025541687903740 === Application of the technical nickel-based alloy Inconel 617 as a material for gas tubes in the primary circuit of the high temperature gas-cooled reactor is limited essentially by the chemical reactions of the alloying elements with the impurities (CO, CO2, H2, CH4 and H2O) in the helium coolant. Depending on the temperature and gas composition, carburization or decarburization as well as oxidation occur. … The results demonstrate that the occurrence of the various reactions is determined essentially by temperature. For a standardized helium gas (HHT-He), three temperature regions can be distinguished between. Below a critical temperature (about 1105 K) the presence of CO can cause simultaneous oxidation and carburization. Above about 1105 K, this reaction does not reverse itself. Rather, oxidation by CO2 and H2O takes place which shows, after a transient state, the same kinetics as those observed in undiluted oxygen-containing gases. At temperatures above about 1205 K, decarburization of the alloy accompanied by the production of CO takes place, leading to severe destruction of the carbide microstructure and therefore limiting the applicability of the material. === The temperature of Turbine Exhaust is 717 C or 990 K which is 115 C less than the critical temperature. ALLAM Cycle uses CH4 as fuel. In case the supplied oxygen enriched CO2 is less than required, the partial burning of CH4 might produce CO. We do not know the effect of simultaneous oxidation and carburization of the 617 alloy by the CO on the strength of alloy 617. Thus, it would be prudent to avoid PCHE made using alloy 617 in ALLAM Cycle. The start-up and shut-down cycles cause fatigue. We could use two PCHE made of stainless steel and use them alternately. We pass the Turbine Exhaust at 30 bar and 717 C and flow 9 getting heated up to 717 C at 310 bar during one duration to be fixed. During this duration, we pass the Turbine Cooling Flow in the other PCHE cooling the PCHE to about 548.5 C. In the next period, the Turbine Exhaust and flow 9 pass through the cooled PCHE while the Turbine Cooling Flow cools the PCHE that has heated to 717 C. Thus the PCHEs alternate between 548.5 C and 717 C. This change in temperature is not as severe as 25 C and 717 C due to start-up and shut-down cycles. Keeping the duration as high as possible reduces the fatigue but increases the possibility of deforming the channels through which flow 9 passes. A Google search on – stainless steel diffusion bonding time hours – was made. One of the results was http://www.dtic.mil/dtic/tr/fulltext/u2/a186875.pdf . This result was a lucky break. The term ‘hours’ occurred in the document not because the bonding time was in hours, but that there was a break in the experimental set up of 4 hours. The bonding was performed uniformly for 30 minutes in all the experiments. The bonding between two surfaces of silver coated SS 316 was attempted. The silver was sputter coated. It did not form bond with the stainless steel. After the experiment conducted at 200 C, 300 C, and 400 C diffusion bond between the silver surfaces did not form at 200 C, but were made at 300 C and 400 C. The applied pressure was 1,379 bar. The melting temperature of silver is 1,233 K. The applied temperatures were 38%, 46%, and 55% of the melting temperature of silver. We are interested in finding out whether passing flow 9 at a pressure of 310 bar at a temperature of 717 C is acceptable. The melting temperature of SS 316 is 1400 C. Thus 717 C is 51% of the melting temperature of SS 316. From https://www.azom.com/article.aspx?ArticleID=2868#_Mechanical_Properties we find that the ‘Proof Stress’ of SS 316 is a minimum of 2,000 bar. Thus 310 bar being just 15.5% of the ‘Proof Stress’ we do not expect any deformation of the PCHE due to the flow 9. We plan to subject the PCHE to temperatures between 548.5 C and 717 C and not 25 C and 717 C corresponding to a cold start. Treating the swing from 548.5 C as a hot start, we believe no fatigue would be caused. For guidance, we consider the start-up and shut-down issues in fossil-fuel base-load power plants. http://www.powermag.com/make-your-plant-ready-for-cycling-operations/?pagenum=1 === Few conventional steam plants were designed to follow load, cycle from minimum to full load every day, or shut down and start up daily, as so many plants are called upon to do these days. … The following discussion applies equally to solid fuel–fired steam plants and natural gas–fired combined cycle plants. … The fatigue damage added to an older baseload power plant causes creep fatigue interaction damage, rapid increase in boiler tube failures, and many other component failures, including turbine generator and balance-of-plant early creep fatigue failures. … In our experience, about 60% to 80% of all power plant failures are related to cycling operations. === http://www.powermag.com/make-your-plant-ready-for-cycling-operations/?pagenum=2 === One approach to reducing cycling damage is to modify the unit’s low-load limit to prevent cycling damage. By reducing the low-load limit on a 750-MW supercritical gas-fired unit from 150 MW to 28 MW at night, it was possible to make the daily cycling load profitable, [From the figure regarding the different types of starts for a steam turbine, we find: 1. Hot Start – 5 hours or less off line 2. Warm start – 5 hours to 40 hours off line and 3. Cold start – 40 hours or more off line] === http://www.powermag.com/make-your-plant-ready-for-cycling-operations/?pagenum=4 === Based on our experience with combined cycle plants, we have found that the largest line item on a plant’s operating and maintenance budget is costs related to cycling. … One reason cycling costs are proportionally higher in combined cycles is that turbine inspection and repair intervals are transitioned to being principally based on numbers of starts rather than operating hours. Therefore, the cost to maintain combined cycle availability is high on a per-unit-of-electricity-produced basis. The cost of replacement parts found damaged during overhauls, or “parts fallout” due to cycling, is often a factor of two or more over the plant life compared with baseload operation. === http://www.powermag.com/make-your-plant-ready-for-cycling-operations/?pagenum=6 === Cycling at the lowest possible ramp rate that satisfies system needs or economics will often reduce the damage rates. … Keep offline time short and the equipment hot to reduce cold starts, especially with the boiler/heat recovery steam generator. === For the base-load power plant designed to run 24/7 we are advised to keep the off line time less than 5 hours so that the restart is a ‘hot start’ causing reduced damage. Thus, we believe keeping 2 Heatric PCHEs and alternating the flows through them would not cause fatigue failures. Such an arrangement avoids using pumps not planned by the team led by Rodney Allam. Based on the PCHE performance curve of December 12, 2013 we found that safe operation of the PCHE at 310 bar is permitted at a temperature of 630 C and not 717 C. The same performance curve gives the maximum temperature even at atmospheric pressure to be slightly more than 800 C. In a design article, https://www.heatric.com/hres/design%20considerations%20for%20compact%20heat%20ex changers.pdf published during 2008, we find: === Fig. 13 shows ASME VIII allowable stresses for 316 stainless steel. Although the allowable stresses are listed up to a maximum temperature of 550 C, the use of 316 is permitted up to 600 C. Allowable stresses are not quoted by ASME VIII for the range 550 – 600 C, but an in-house programme is in progress at Heatric for design in this temperature range. === It is possible that the performance curve of December 12, 2013 was based on the standards approved possibly in 2012. As on August 2013, we find: http://www.worldstainless.org/Files/issf/non-image-files/PDF/Atlas_Grade_datashe et_-_all_datasheets_rev_Aug_2013.pdf === Page 14 of 57 Heat Resistance Good oxidation resistance in intermittent service to 870 C and in continuous service to 925 C. Continuous use of 316 in the 425-860 C range is not recommended if subsequent aqueous corrosion resistance is important. === In the case of ALLAM Cycle, moisture is present in the turbine exhaust only at a pressure of 30 bar and temperature of 717 C. There is no moisture in flow 9 and thus there is no need to worry about subsequent aqueous corrosion. We infer that 316 could be used up to 870 C under intermittent operation. Thus oscillating between 548.5 C and 717 C should cause no difficulty. In case the performance curve is revised to permit 925 C instead of 811.58 C as given in the 2013 performance curve, the permissible temperature at 310 bar becomes (925/811.58) x 630 = 718 C. Thus, the design by Rodney Allam giving flow 9 to be at 310 bar and 717 C is correct. We still recommend intermittent operation of two PCHEs in case the perfect performance expected by Rodney Allam does not materialize. We find the following increasing our anxiety regarding the ‘First Fire’ at La Porte, TX. http://docs.house.gov/meetings/SY/SY20/20170503/105930/HHRG-115-SY20-Wstate-Dimm igW-20170503.pdf === May 3, 2017 Only the turbine and combustor are novel, but the turbine relies on proven technologies from both the gas and steam turbine industries. The combustor, though, did require R&D by 8 Rivers and Toshiba, and it has since been proven at the 5MW scale. === The combustor was tested successfully during July, 2013. https://www.toshiba.co.jp/about/press/2013_08/pr0101.htm === 1 Aug, 2013 TOKYO—Toshiba Corporation (TOKYO: 6502) today announced the successful testing of a first-of-its-kind combustor to be used in a breakthrough thermal power generation system that produces low-cost electricity and little-to-no air emissions. Step-by-step testing of the new combustor was initiated at a facility in California in January 2013, to confirm and demonstrate its operation within the new generation system's required environment: never before demonstrated high operating pressure and temperature, plus a unique combustion mixture of oxygen, natural gas and a high concentration of CO2. In July, the test program broke new scientific ground by confirming stable operation at the system's target pressure of 300 atmospheres, fully confirming Toshiba's design. === The above press release from Toshiba does not mention the power of the combustor. It is inferred from the following quote. The web page given by https://www.sciencedirect.com/science/article/pii/S187661021300221X has a PDF download link. The download gives the following PDF. We quote from the PDF. https://ac.els-cdn.com/S187661021300221X/1-s2.0-S187661021300221X-main.pdf?_tid= 22d64123-3c51-487c-9078-6aee8bd5b82d&acdnat=1523635532_ded0c6d324b1f7f78065f491d 079a3b5 === Page 9 of 15 Recent developments in combustors for heavy duty gas turbine have been focused on decreasing NOx emissions. This effort has led to pre-mixed combustion, in which fuel is mixed with air before combustion to enable lower temperature combustion. The disadvantage of pre-mixed combustion, however, is that it causes system vibrations, called dynamics, due to flame instability. In this regard, the present NET Power system is advantageous for combustion because it eliminates NOx while enabling adoption of simpler and more stable diffusion combustion. The system is also able to use proven cooling technology, such as back side convection cooling, due to the moderate temperature of combustion and the high cooling capability and high availability of carbon dioxide. Toshiba is planning to conduct a high pressure rig test of a NET Power combustor, scaled down by 1:5, at the end of 2012. That test system, …, will reveal combustion characteristics during simulations of ignition, load-up and operation in order to confirm combustion stability performance, combustion dynamics, temperature distribution, blow-out and other features. === From August 1, 2013 press release of Toshiba, we understand that the 5 MW combustor is successfully tested. As the La Porte, TX plant is for 25 MW, we need 5 combustors for the single turbine. From the following quote claiming that the La Porte plant is fully built, we know that Toshiba has delivered the combustor, possibly one for 25 MW. https://www.eenews.net/stories/1060071081/print === January 16, 2018 The 50-megawatt demonstration from NET Power LLC in La Porte, now fully built after breaking ground two years ago, is the world's largest attempt to use carbon dioxide rather than steam to drive a turbine. … But first, the company has to get through initial testing in the next few months, including firing the demo plant's combustor, powering the turbine and connecting to the Texas grid. … The two biggest areas of concern are whether the combustor and turbine in La Porte will operate as specified and whether the heat exchanger that cools flue gas performs well, he said. Even though similar machinery has been tested elsewhere, it has never been tested exactly in this way, said Herzog. … The combustor being tested in La Porte will be the same one used at scale — there will just be more of them. === When building a commercial scale 300 MWe plant, if Toshiba plans to use combustors of 25 MW, they need to use more than one combustor in parallel for every turbine. It is our estimate that it would be safe to use one combustor per turbine to avoid problems of matching the pressure of the flame between all compressors connected to a turbine. Toshiba having tested a 5 MW combustor in 2013 has built and delivered a 25 MW combustor before 2018. Thus, if Net Power and Toshiba still insist on abandoning the 25 MWe market and concentrating on the 300 MWe market, Toshiba could build larger combustor so that each turbine is fueled by a single combustor only. Bright future: The following quotes indicate that the ALLAM Cycle has a bright future indeed. http://www.grandforksherald.com/news/4405404-eerc-project-wins-6-million-federal -funding === Feb 17, 2018 The carbon capture effort comes alongside other research at the EERC intended to make coal and natural gas-fired power plants more efficient using a technological approach known as the Allam Cycle, a method that has carbon capture benefits. Earlier this year, that line of research at UND was awarded a separate $700,000 grant from the DOE. === https://www.technologyreview.com/lists/technologies/2018/ === This story is part of our March/April 2018 Issue The world is probably stuck with natural gas as one of our primary sources of electricity for the foreseeable future. Cheap and readily available, it now accounts for more than 30 percent of US electricity and 22 percent of world electricity. And although it’s cleaner than coal, it’s still a massive source of carbon emissions. … The company is in the process of commissioning the plant and has begun initial testing. It intends to release results from early evaluations in the months ahead. === https://slate.com/technology/2018/03/carbon-capture-and-storage-a-technology-to- fight-climate-change-is-becoming-a-reality.html === MARCH 06, 2018 Enter Net Power, a startup with a new carbon-capturing natural gas-fired electricity generation technology. Net Power’s novel “Allam cycle” technology fundamentally redesigns how fossil-fuel electricity generators have traditionally worked in order to avoid the separation challenge and produce an easily separable exhaust of pure carbon dioxide and water. The result should be a power plant that can generate electricity as efficiently as the leading existing gas-fired electricity generators, all while capturing 100 percent of its emissions at zero additional cost. Net Power is currently commissioning its pilot plant near Houston. If it performs as advertised, this technology could smash the idea we need to pay more for clean energy. Net Power, like all large-scale and complex technologies, will have additional costs for initial projects, but they will go down as the developers learn from each successive project. The tax credits will provide the extra support needed to enable the first full-scale commercial plant to be built and to accelerate subsequent deployment. === All the above quotes indicate that the future for ALLAM Cycle and CAM ALLAM Cycle is bright. It all depends on the successful ‘First Fire’ of the demonstration plant at La Porte, TX. Will it happen? Credibility on the line: From our analysis we find that the Heatric PCHE has no flaw. We perceived a flaw because of the old PCHE performance reported in the Heatric web. In our anxiety to learn about the successful ‘First Fire’ we look for reasons of delay. In the absence of possible technical shortcoming, we consider financial aspects now. https://www.eenews.net/stories/1060071081/print === January 16, 2018 Overoptimistic or not, Brown said his goal is to have a contract signed by the end of the year to build the full-scale plant, so operations can begin by 2021. … The company has narrowed down sites for that to eight or so around the world, including two in Texas, one in North Dakota, and several in the Middle East and the United Kingdom, said Brown. … NET Power's experiment is being watched closely. … the demonstration received about £7 million pounds from the U.K. government. … The La Porte project is also backed by about $140 million of industry money, including $90 million from energy giant Exelon Corp. and $50 million from engineering firm Chicago Bridge & Iron Company (CB&I). === The La Porte, TX demonstration is for a 50 MWth plant. Already it has raised ₤7 million [ equals 7,000,000 x 1.42 USD on April 11, 2018 = $9.94 million ]. Another $140 million is also included. If ‘First Fire’ succeeds now, we could say, $149.94 million is the cost of a 50 MWth plant using the ALLAM Cycle. Let us say $150 million is the cost of a 25 MWe plant using the ALLAM Cycle. This works out to a rate of $6,000 per KW. We extract the following fromhttps://www.eia.gov/outlooks/aeo/assumptions/pdf/table_8.2.pdf .
Coal with 90% CCS of 650 MW capacity to be completed by 2021 costs $5,628 per KW.
Advanced combined cycle with CCS of 340 MW capacity costing $2,175 per KW is expected to be ready by 2020.
Fuel cell technology for 2020 with 10 MW capacity might cost $7,132 per KW.
Advanced nuclear of 2,234 MW capacity costing $5,946 per KW is expected to be running by 2022.
At $1,553 per KW a distributed generation base plant of 2 MW capacity is expected to run by 2020 and
Biomass of 50 MW capacity to be ready by 2021 would cost $3,732 per KW.
The demonstration plant with 25 MW capacity costs $6,000 /KW and happens to be the costliest except for the fuel cell technology. For comparison, we take the biomass technology that has a capacity of 50 MW and costs just $3,832 /KW. What about the full scale 300 MW ALLAM Cycle plant? https://www.eenews.net/stories/1060071081/print === An initial commercial-scale plant could cost $500 million and have higher capital costs than a traditional natural gas plant … === The expected commercial ALLAM Cycle plant would have 300 MWe capacity and would cost $500 million / 300,000 KW = $ 1,667 /KW. The commercial plant compares favorably with ‘Advanced combined cycle with CCS’ at $2,175 /KW and ‘Biomass’ at $3,832/KW. But the commercial plant requires long distance transmission lines which are to be avoided in future. Avoiding the transmission line, the ALLAM Cycle plant with 25 MWe capacity is costlier than biomass unless subsequent installations of the 25 MWe plant could be done at a substantial lower cost. The ALLAM Cycle demonstration plant uses just 5 acres for the plant. For comparison, we consider a biomass plant built by Toshiba in Japan. http://biomassmagazine.com/articles/14336/toshiba-fires-up-50-mw-biomass-power-p lant-in-japan === The plant will be fueled with palm kernel shells (PKS), the highly fibrous shell fractions left after extracting oil in palm oil mills. SPAC will import 0.2 million tons of PKS, mainly from Indonesia via Miike port in Omuta. It will be stored in a newly-built roofed yard with the area of about three soccer fields and a storage capacity of 30,000 tons. === Let us find the area of a soccer field. http://www.stack.com/a/how-many-acres-is-a-football-field === High school, college and NFL football fields are the same size. A standard football field is 120 yards long. The playing field is 100 yards (300 feet) long, and each end zone is 10 yards (30 feet) deep. The field has a uniform width of 53 1/3 yards (160 feet). If you calculate the entire area of a football field, including the end zones, it works out to 57,600 square feet (360 x 160). One acre equals 43,560 square feet, so a football field is about 1.32 acres in size. === From the value of 1.32 acres for a soccer field, we find the area of storage in the Toshiba built biomass power plant is 3.96 acres. We would be right if we assumed that the area for a comparable biomass power plant to the demonstration plant at La Porte, TX would be more than 5 acres. Even though we might pay for more in area, the biomass power plant has no worry about carbon capture and storage and it is definitely superior in environmental benefit. Unless the ‘First Fire’ of the ALLAM Cycle demonstration plant in La Porte, TX is completed successfully soon, world might lose interest in the ALLAM Cycle itself. Is Net Power waiting for the US Administration to start supporting the construction of commercial ALLAM Cycle power plants? Is it postponing the ‘First Fire’ at La Porte, TX until it hears positively from the current administration? We would like to remind ourselves that in 2016 the general assumption was Hillary would be President and thus Climate Action would have received a strong support from the Federal Administration. https://www.eenews.net/stories/1060071081/print === If all goes well, developers are planning to build an additional 300-MW plant that is emissions-free, without polluting smokestacks. … "I am confident that we have created something that will allow the world to meet every climate target without having to pay more for electricity," said CEO Bill Brown in an interview. … Overoptimistic or not, Brown said his goal is to have a contract signed by the end of the year to build the full-scale plant, so operations can begin by 2021. … The company has narrowed down sites for that to eight or so around the world, including two in Texas, one in North Dakota, and several in the Middle East and the United Kingdom, said Brown. … NET Power's experiment is being watched closely. … NET Power has been cited as an example of industry making innovations on its own, considering it did not receive a dime of U.S. government money. Still, Brown said government support could be pivotal in whether a larger plant gets built. … "It would make it a great deal easier," he said === We hope Net Power raises private capital and continue rather than hoping the present administration, which has already started campaigning for the 2020 election, would hurt its fossil fuel lobby and help Net Power. Why? If Net Power succeeds in generating electricity at comparable cost to the best existing fossil fuel plant with 100% CCS, all the current leaders of conventional and costly CCS would lose. Moving forward, after supporting Net Power this administration might not raise enough funds for the 2020 election. The companies that would benefit from the success of Net Power include Heatric of UK and Toshiba of Japan. The current administration is very sensitive to the trade imbalance as of now. If virtually all the future natural gas plants all over the world would be built following the ALLAM Cycle, most of equipment profits would accrue to UK and Japan. Such a future does not make ‘America Great Again.’ In view of this aspect also, we do not expect this administration to ever give a grant to Net Power. We repeat, it would be wise for Net Power to successfully complete the ‘First Fire’ at La Porte, TX soon and move ahead with private capital or grant from countries concerned about climate change. Conclusion: With respect to the Recuperator, if our suggestion to operate two PCHEs in alteration is not feasible, the fourth stage PCHE could be replaced with the time tested shell and pipe heat exchanger. We wish the use of shell and pipe heat exchanger could be avoided. We understand that as of now none of our suggestions could be implemented at La Porte, TX. To move the pumps that pressurize flows 8 and 12 to pressurize the flows 9 and 13 after the Recuperator would cause major rework in piping. To replace the high temperature PCHE with shell and pipe heat exchanger also would need major reconstruction. So also adding a second Heatric PCHE to operate two PCHEs in alternating mode would cause major rework. Thus none of our suggestions are implementable without causing delay in ‘First Fire.’ To move ahead with the ‘First Fire’, we suggest the engineers of Net Power calculate the transient time within which the high temperature PCHE would remain stable and conduct the ‘First Fire’ for a shorter duration within the safe operating time. Even if the demonstration plant could work for 1 hour successfully, the ‘First Fire’ is a success. The subsequent 25 MWe ALLAM Cycle plants could be designed for 24/7 operation taking advantage of our suggestions. We hope that Net Power would concentrate on 25 MWe ALLAM Cycle plants and install them in thousands all over the world starting 2018 itself, rather than waiting for 2021 to successfully demonstrate the 300 MWe plant which in any case would be of no use once the transmission lines fail in the next hurricane. As of now the first-of-a-kind 25 MWe plant at La Porte, TX costs $6,000 / KW. We believe that more implementations of the 25 MWe plants would approach the projected commercial plant cost of $1,670 /KW making the ALLAM Cycle more attractive than even the biomass plant. We conclude by quoting Walker Dimming, Principal of Net Power. http://docs.house.gov/meetings/SY/SY20/20170503/105930/HHRG-115-SY20-Wstate-Dimm igW-20170503.pdf === A first-of-its-kind commercial-scale facility will need to operate commercially in the market in order to be developed, and yet it will be a significantly more expensive project than the second facility of its kind will be. First commercial-scale projects suffer from a number of challenges that are unique to being a first-of-a-kind. Because they are not yet mature technologies with full customer order-books, they will not receive the benefit of a supply chain that has maximized its efficiencies and become fully competitive. Every piece of equipment in the plant is likely to be more expensive than in an “Nth-of-a-kind” facility; the design of the plant will not yet have been fully optimized; there will be large engineering costs unique to a first-of-a-kind design; and contingencies are typically added across the development process for the increased risk of the project. === We searched on April 16, 2018 to check whether the ‘First Fire’ has happened at La Porte, TX. We found the following indicating that it is yet to happen. https://wri-indonesia.org/en/blog/taking-greenhouse-gases-sky-7-things-know-abou t-carbon-removal === 02 April 2018 A new power system called the Allam Cycle is also something to watch. The technology makes carbon capture part of the core electricity-generation process. NET Power is using the technology in the first-ever zero-emissions natural gas demonstration plant in La Porte, Texas, which is expected to begin supplying power to the grid in 2018. If it works, the Allam Cycle facility will capture all the carbon dioxide it produces, and is projected to be cost-competitive with conventional natural gas power systems once the technology matures. This demonstration plant could therefore have a transformative effect on the power system, and incentivize investments in critical infrastructure like geological storage, which will ultimately help bring down the cost of carbon removal. === We would appreciate hearing about the ‘First Fire’ of the ALLAM Cycle plant at La Porte, TX.
The earlier links in this series are: